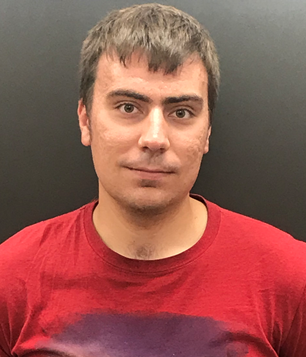
"Capturing experimental properties of metal oxides in efficient computational models"
Metal oxides will be critical to the function of a new generation of catalysts, electronics, and photochemical devices to help solve pressing global problems related to clean energy generation and storage. While the properties of these materials in bulk have been understood for decades, it is often disorder of the perfect crystal lattice that gives rise to technologically relevant characteristics. Calculating the properties of such disordered systems is nontrivial, and requires true-to-experiment computational representations that reliably capture the real system. This talk reviews recent efforts to develop modeling criteria for a range of prototypical disordered metal oxides, including point defects, interfaces, and amorphous solids to build a comprehensive understanding of their structure-function relationships. Through systematic study of parameter space, it is shown how popular modeling approximations, such as periodic boundary conditions, can give rise to computational artifacts that can be identified and eliminated through comparison to experimental data. Furthermore, it is demonstrated how proper model selection can lead to new chemical insights through case studies of multiscale oxygen diffusion through doped SrTiO3 and amorphous TiO2 surface generation. Such model development is critical for repositioning computation as a predictive tool for rational design of new materials and their properties.